An ancient crocodile’s last meal might never have come to light were it not for researchers deciding to scan the rock-embedded fossil with a beam of neutrons. The scientists had set out to see if neutrons—the building blocks of atomic nuclei, along with protons—could offer better images of fossils than x-rays and made the startling discovery that a croc in the Cretaceous period had eaten a previously unknown species of juvenile ornithopod dinosaur before it died.
The archeological surprise is just one of many feats that would be difficult or impossible without subatomic particles. These tiny bits of matter have long been interesting to physicists seeking to understand the underlying laws of nature, but they are proving to have much more practical uses as well. Researchers are increasingly turning to protons, neutrons, muons and neutrinos as tools for precisely targeting tricky tumors, probing fossils and volcanoes and revealing the hidden structures of Earth, among an ever expanding list of applications.
Cancer-Killing Missiles
Protons are abundant. Along with neutrons and electrons, they’re components of the atoms that make up us and everything around us. When pulled out of atoms and sped up in particle accelerators, they become precise cancer-fighting tools that are safer and more effective than more common x-ray and gamma-ray treatments, according to Nancy Lee of Memorial Sloan Kettering Cancer Center in New York City.
“Protons are always better,” Lee says. The logistic challenge of providing the therapy to people at a reasonable cost is the primary reason patients still turn to other options. “Right now the waiting lists [for proton beam therapy] are well over a month,” she says.
Proton therapy for cancer was pioneered in the 1950s. But by the early 2000s, fewer than 10,000 people had benefited from it. In the two decades since, the number has exploded to about 200,000 patients worldwide. Treatment facilities have also multiplied, from a dozen or so at the beginning of the century to more than 100 today.
Doctors can tune beams of protons to precisely destroy specific targets, usually cancerous tumors, without harming nearby organs—unlike x-rays and gamma rays, which have historically been the go-to beams for cancer therapy. These two varieties of photons, or particles of light, damage healthy tissue both in front of and behind the tumor that’s the intended target. Proton beams, on the other hand, do comparatively little damage to tissue in front of a tumor and none to tissue behind it, thanks to the way protons lose energy as they pass through material, including the human body.
When an accelerated proton enters your body, it loses some energy because of collisions with the atoms in your cells. But it doesn’t distribute energy along its path the way photons do. Instead a proton releases its energy in one quick burst after traveling a distance that depends on the proton’s initial energy. By adjusting the energy of the protons coming out of an accelerator, a doctor can choose the penetration depth that will deliver them directly to a target.
It’s a bit like sending a cancer-killing missile into a tumor: some damage will result from the missile’s path through the body, but the burst at the end is much more destructive.
The proton’s heft and electric charge are what allows the precise penetration. Theoretically, particles that are heavier still would be even more precise. Researchers are investigating radiation therapy that relies on electrically charged carbon atoms, which contain six protons and six neutrons, making them much more massive than individual protons. But Lee suspects that protons are good enough and will prevail as the more developed option for the foreseeable future. And she believes proton therapy will soon get even more effective, thanks to briefer, more intense proton beam treatments known as FLASH therapy, which are in the works.
Neutron Scanners
The Cretaceous croc that had been snacking on a dinosaur exemplifies the other growing uses of fundamental particles. “I was blown away and didn’t believe it at first,” says Matt White, a paleontologist at the University of New England in Australia, who co-authored a paper in Gondwana Research describing the discovery. “Fossilized stomach contents are extremely rare, especially [for] crocodiles, who have the most potent stomach acids in the animal kingdom.” Without neutron tomography, as this kind of mapping is known, nothing short of breaking the fossil apart would have revealed the unfortunate dino.
Neutrons are similar in mass to protons but lack electric charge. That allows them to pass much more easily through matter. Neutrons have a history of use in cancer treatment but have lost ground to proton beams since the 1990s. It’s their potential for imaging that is on the rise these days.
Neutrons pass through lead and other dense materials that stymie x-rays, providing an interior view of engines, fuel cells, mail packages and even nuclear warheads without the need to cut them apart. The leading sources of imaging neutrons include nuclear reactors and particle accelerators, which aim high-energy protons at targets to knock neutrons loose from atoms of heavy metals such as tungsten or mercury.
These particles have been in wide use for decades, but new imaging techniques are expanding the field to the study of rocks and sediments for geoscience, nondestructive analyses of art and antiquities, and even living plants. Mirrors adapted from NASA’s space-based telescopes are on the verge of expanding neutron imaging capabilities on very small scales. “We’re going to soon, hopefully, realize the first practical neutron microscope,” says Daniel Hussey, a physicist at the National Institute of Standards and Technology.
It’s tough to make lenses for neutrons, but mirrors can focus the particles, provided they’re made of a material that reflects neutrons well. “We launched a project, in collaboration with [the Massachusetts Institute of Technology] and NASA Marshall Space Flight Center, to convert the nickel foil mirrors that are used for x-ray telescopes,” Hussey says. Nickel happens to be a good reflector for neutrons, which he predicts will allow the team to focus intense neutron beams to scales of a millionth of a meter. The result will be rapid, high-resolution neutron images of structures a fraction of the size of a red blood cell and a powerful new window on the microscopic world.
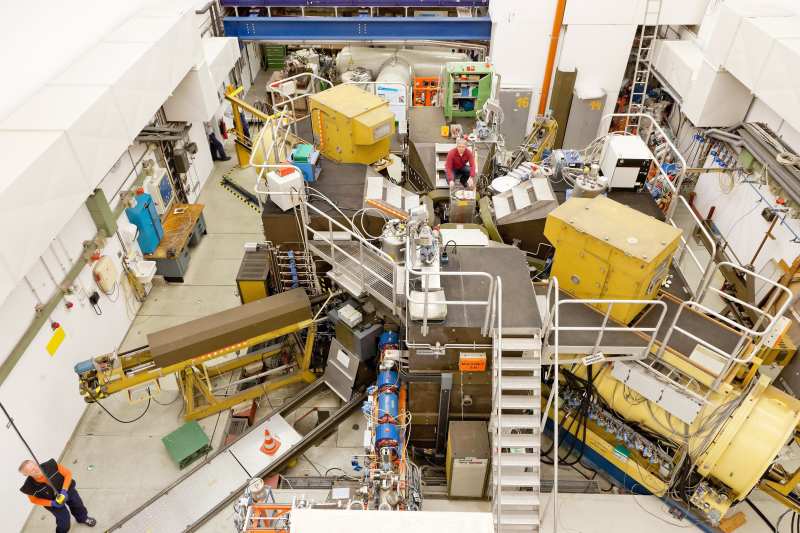
Practical Particle Illumination
Earth is awash in particles coming from high in the atmosphere. Muons, the heavier cousins of electrons, pass through our bodies at a pace of thousands per minute. Ghostly neutrinos are even more numerous—100 trillion glide through the average-size person every second. Although we’re typically unaware of the particle showers, for the past few decades, scientists have begun to exploit them for a host of imaging applications in archeology, geology and even national security.
The muons and many of the neutrinos that make it to the surface of the planet begin with cosmic rays that ram into the upper atmosphere. The rays are primarily protons that come from the sun or originate in deep space. Whatever their source, they create a burst of particles when they smash into atoms in the air. Most of the particles, including electrons, photons and short-lived pions, either break down or get scattered and absorbed by atmospheric gases. That leaves muons and neutrinos to continue down to the surface. It’s their inherent survivability, when compared with other cosmic ray debris, that makes muons and neutrinos interesting as probes of structure within objects.
“Muons are really ideal for a range of sizes spanning from meters to [a] few kilometers,” says Andrea Giammanco, a particle physicist with the Catholic University of Louvain in Belgium. They are handy for probing the innards of things as small as a rain barrel and up to the scale of a skyscraper. These techniques have been known as muon tomography for three-dimensional imaging and muon radiography for two-dimensional imaging, but the term muography is more widely used for both techniques these days.
The first practical application of particles from cosmic rays dates back to the 1950s, when Australian engineers rolled a muon detector along a tunnel that would eventually guide water to the power station associated with the Guthega Dam in New South Wales. Fluctuations in the count of atmospheric muons that made it through provided a measure of the thickness of the material lying on top of the tunnel.
University of California, Berkeley, physicist Luis Alvarez raised the muography bar when he led a team to search for hidden chambers in one of the pyramids of Giza in Egypt in 1968. By measuring the atmospheric muons that passed through the stone, they found there were no unknown chambers. Although disappointing from an archaeological point of view, it showed the muons from cosmic rays could unveil the inner structure of a pyramid without disturbing a single stone.
Despite these successes, scanning based on cosmic ray descendants largely went on hiatus until 2003, when the number of muon imaging papers and experiments took off.
“We figured out how to use the scattering of cosmic ray muons,” says Konstantin Borozdin, who was part of a Los Alamos National laboratory group that published a paper in the journal Nature that helped relaunch muon imaging technology. By looking at how muons scatter as they pass through a material instead of just checking to see how many are absorbed, Borozdin and his colleagues increased the resolution of the images they could create. The technique came in handy for monitoring the interior of the Fukushima power plant after the 2011 disaster. It has since led to new systems for searching trucks for drugs, weapons and other contraband, even items hidden in containers and among materials that would block x-ray scanners.
The most recent applications of muography include tracking the flow of magma under volcanoes, monitoring tides and analyzing the interior of structures to understand how bridges, buildings and wind turbines age. Just about anything too big or dense to x-ray and too small to study with seismic waves is fair game for muon imaging.
A Look inside Earth with Neutrinos
When it comes to Earth as a whole, cosmic-ray-produced neutrinos are on the verge of providing information that no other geologic methods can offer. Neutrinos probe far deeper than muons because they lack electric charge; they are not swerved off their paths by electrically charged protons and electrons in atoms. They stream with little effect through anything much smaller than the planet, but they can potentially provide a look inside Earth that no other technique can match.
“There are neutrinos all around the Earth coming in from every direction at the same time, so we can get a lot of data,” says Rebekah Pestes of Virginia Tech, who has analyzed the potential for future neutrino detectors to scan the planet.
At the moment, seismology currently offers a better picture of Earth’s interior. But Pestes suspects that neutrinos could eventually offer a detailed view of the planet’s insides, thanks to an unusual feature: Neutrinos transform as they travel, oscillating among three types known as “flavors.” The rate of their oscillation depends on the chemical composition of the matter they travel through. “Oscillation tomography [can] provide a more direct way to measure the composition of the Earth,” says Paris City University professor Véronique Van Elewyck.
Neutrinos also flow directly from nuclear reactors and radioactive waste, which has led to some proposals to use them to verify international nuclear agreements. Detectors specifically designed with nuclear submarines in mind would provide diagnostics and monitoring without requiring access to secured areas inside the craft. This idea is still preliminary, however.
These uses represent just some of the growing number of ways particles can come in handy. The unfortunate dinosaur in the belly of a Cretaceous croc was one unexpected discovery that came of the expanding particle toolbox. It won’t be the last.